Insecticide resistance is a global problem that poses mounting challenges to human health. Insects’ ability to quickly adapt to chemical interventions renders products ineffective and undermines pest management efforts in both agriculture and public health. This compromises our capacity to produce a safe and affordable food supply while impairing our ability to safeguard society against crippling or even fatal vector-borne disease.
From a high level, there is no great mystery as to how resistance occurs. The overarching concept of selection pressure is well understood and relatively straightforward. Effectively combatting the problem is something else entirely, and those discussions must be rooted in the understanding that resistance is not founded on one single biological process. Rather, there are multiple mechanisms at play within the sphere of insecticide resistance, each of which are brought about by the same root cause: use or overuse of chemical controls.
Resisting Resistance Series:
Before examining the various types of insecticide resistance mechanisms, it is important to understand how various classes of insecticides work. Following is a brief overview.
Insecticide Modes of Action
Mode of Action (MoA) is a term used to describe the functional change that occurs at the molecular or cellular level when an organism is exposed to a substance, typically a substance designed for control. MoA is important for the understanding of pesticide resistance.
Resistance develops when an organism adapts through genetic mutations that render a given MoA less and less effective. In the case of insecticides, these mechanisms typically involve the interaction between insecticides and the functional proteins they target within the insect, more specifically, functional proteins that govern an insect’s neuromuscular activity, its growth, respiration, or digestive system (see sidebar).
Protein compounds serve important functional roles within any living organism by regulating bioelectrical activity as well as biochemical reactions in and around cells. Insecticides work through manipulation of processes dependent upon three protein categories: 1) ion channel proteins that facilitate communication of signals across the insect’s neuromuscular system; 2) metabolic enzymes that act as catalysts for biochemical reactions within cells; and 3) protein receptors on cell membranes that respond to external signals to induce activities within the cells. Insecticides target these compounds and processes.
Neuromuscular Disruptors
The most common type of insecticides are those that affect ion channels. Ion channels are present in all cells membranes and regulate the bioelectrical impulses that travel rapidly across the insect’s central nervous system to control its muscles and joints. The opening and closing of these channels (or gates) is controlled by a variety of proteins. Insecticides that target ion channels are known as neuromuscular disruptors. Depending on the molecule, neuromuscular disruptors can either inhibit bioelectrical impulses or hyperexcite them, with devastating effects.
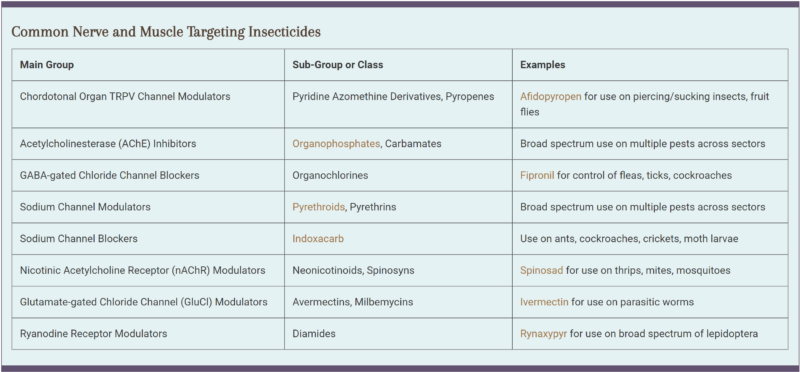
TRPV (transient receptor potential vanilloid) is a group of calcium selective ion channels related to sensory activity. In insects, these stimuli arise from their antennae or joints. TRPV channel insecticides cause continuous firing of these signals, which leads to lack of muscle coordination within the insect. This prevents the insect from feeding, and ultimately leads to starvation and death. Another group, sodium ion channels, regulate cells’ action potential–rapid changes in voltage that serve to contract muscle cells. Sodium channel modulators are insecticides that keep these channels open, causing repetitive firing of nerve cells, hyperexcitation of muscle cells, and rapid expenditure of stored energy within the insect. Sodium channel blockers are insecticides that halt the passage of these same impulses across muscle and nerve cells, leading to insect paralysis.
Other groups of neuromuscular disruptors interfere with intercellular communications in similar ways. Acetylcholine, Gamma-aminobutyric acid (GABA), and glutamate are important neurotransmitters that allow or prevent impulses from bridging the gap between neurons in the central nervous system. Insecticides affecting these ion channels disrupt communication by blocking or exciting nerve-to-muscle impulses across synapses. This may lead to paralysis or convulsions within the insect, leading to death.
Growth and Development Disruptors
In addition to those that affect the insect’s neuromuscular system, still other insecticides target insects’ growth and development processes. Arthropods are characterized by a protective exoskeleton that serves as framework for their musculature. The exoskeleton also houses and protects their organs and soft tissues. Between their larval and adult stages, insects go through growth phases (instars) that involve molting, or shedding, of the exoskeleton to make way for new bodily growth. Insects’ exoskeletons are composed mainly of a naturally occurring, long-chain biopolymer called chitin, which provides the insect with its rigid, yet elastic outer shell.
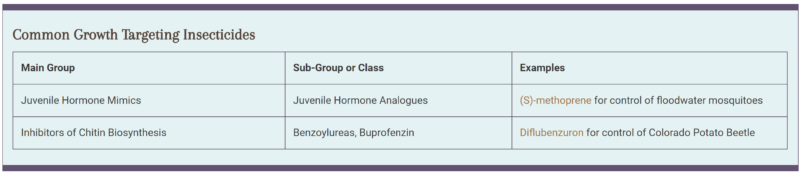
A number of insecticides inhibit chitin production or the process of molting itself, leaving the insect deformed or perpetually immature. These processes are all controlled by insect hormones.
One such class of insecticides focuses on nuclear hormone receptors (NHRs). NHRs are a group of proteins that regulate the expression of genes involved in molting and the development of adult insect structures. The insect hormone ecdysone, for example, plays an essential role in molting. Insecticides that activate ecdysone receptors cause a premature and incomplete molt that results in insect death. Conversely, insecticides that mimic juvenile hormones suppress the insect’s development of adult structures, keeping the insect in an immature state and causing death.
Acetyl CoA carboxylase (ACCase) is an enzyme responsible for the biosynthesis of fatty acids necessary for insect growth and molting. ACCase inhibitor insecticides interrupt this process, also resulting in death. Chitin synthesis inhibitor insecticides operate by interfering the production of chitin, weakening and softening the exoskeleton resulting in deformed extremities and sexual organs, causing the insect to perish.
Insecticides Targeting Respiration and the Midgut
Like all living organisms, insects depend on respiration to release the energy that powers their living processes. At the cellular level, respiration involves the oxidization of fats and sugars into carbon dioxide and water. A specialized organelle within the cell called the mitochondrion captures and stores energy produced from this process in the form of adenosine triphosphate, or ATP.
Cellular respiration is a complex process that uses multiple enzymes, in sequence, to create and conserve ATP. Insecticides that affect one or more links in this process essentially starve the cells of energy, resulting in paralysis and eventual death.
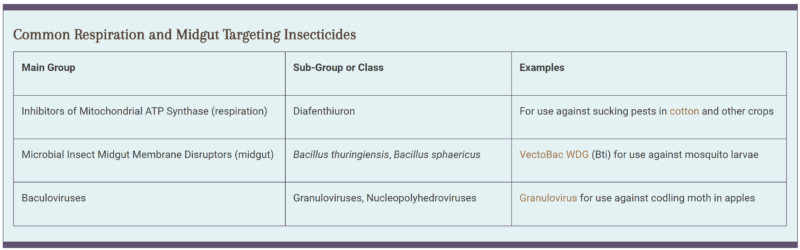
Two types of bioinsecticides–the bacteria Bacillus thuringiensis spp. (Bt), and baculoviruses–function by targeting the midgut of their insect hosts. Once ingested, Bt insecticides contain specialized proteins that break down and breach midgut cell lining, leading to lysis and death. Bt spores also germinate in their hosts, continuing the bacteria’s life cycle. Baculoviruses are viral DNA strands specific to insects that also invade via ingestion. Once inside the midgut, these DNA strands take over cell functions and cause a lethal infection within the pest.
It should be noted that given it’s complex MoA, insect resistance to Bacillus thuringiensis spp. israelensis (Bti) has never been observed in the field despite nearly 40 years of use.
Insecticide Resistance Mechanism Types
As seen in the previous examples, insecticides function by adversely affecting various biochemical and/or bioelectric life processes in the target organism. The challenge lies in the fact that gene mutations resident within the insect population can lessen or even neutralize these effects. Resistance to a given chemical control occurs when naturally resistant individuals survive and carry those mutations forward. Over time, the relative proportion of the population carrying this mutation increases, if the original selecting agent, in this case the chemical, continues to persist in the environment, resulting in the onset of resistance.
To further complicate the matter, not all gene mutations operate in the same manner. The ways in which these mutations counteract the intended control are called insecticide resistance mechanisms. All told, there are four types of resistance mechanisms that have been observed in arthropods. The first three are known as physiological resistance mechanisms–metabolic resistance, target-site resistance, and penetration resistance. A fourth is known as behavioral resistance. The first three involve biochemical adaptations within the target while the latter involves a behavioral change in response to the presence of chemical controls.
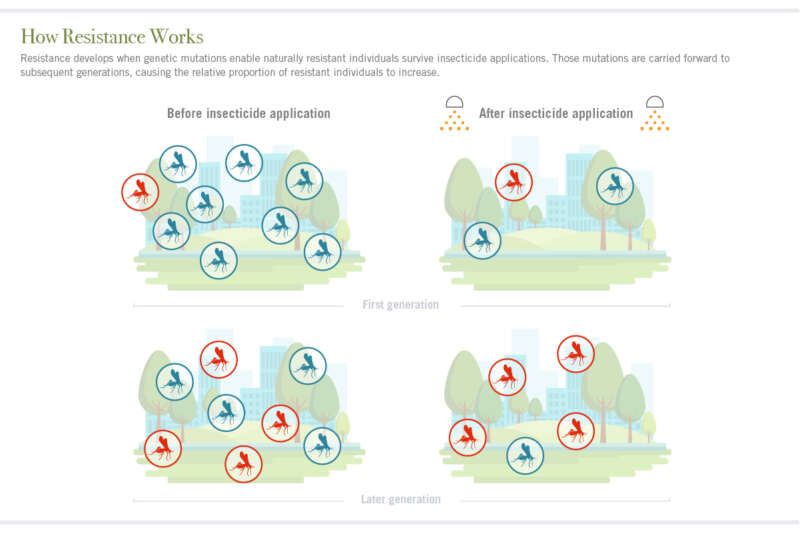
Metabolic resistance is the most common of these mechanisms and refers to a process whereby the gene mutation provides individuals the ability to process the toxin at a much higher rate than the susceptible population. While the chemical may have the desired effect initially, these individuals are able to render the toxin inert, destroy it altogether, or discharge it as waste. Just as enzymes play a key role in many insecticide MoAs, resistant insects can use specific enzymes to break down insecticides, often over a very wide range.
Target-site resistance is a mechanism whereby the mutation prevents the insecticide from binding or interacting at its intended site. This reduces or eliminates the toxicological effect of the chemical within the insect. A third physiological mechanism is known as penetration resistance. While some insecticide must be ingested by the insect to be effective, many are absorbed through the cuticle. Penetration resistant individuals have mutations that slow or prevent absorption of the control chemical into their bodies. Penetration resistance is often complementary to metabolic or target-site resistance.
Behavioral resistance refers to adapted, avoidance behavior by the insect, driven by an avoidance gene, most commonly in females. Behavioral resistance is a growing area of research and has been observed in many insect species.1 Such behavior may include avoiding areas where the insecticide is present, a change in feeding patterns, or avoiding egg laying in pesticide-treated areas. In agriculture, insects may avoid feeding on plants treated with an insecticide or may feed on the underside of treated leaves. In vector control, cockroaches have been observed to avoid baits and mosquitoes have been observed to change their indoor feeding patterns to daylight hours when humans are not protected by insecticide-treated nets.
References: Insecticide Resistance Action Committee Tutorial on Mode of Action Mechanisms; Mode of Action Classification[/vc_column_text][/vc_column][/vc_row]