Tens of thousands of unexplored forest plants may hold the key to new therapeutic drugs for curing a planet rocked by pandemics and new diseases.
They called it Project 523 after its start date, 23 May, 1967. Back then, it was classified as a top-secret state mission, blessed by Mao Zedong, Chairman of the Chinese Communist Party. The Vietnam War was in full swing, and China’s ally Ho Chi Minh, the Prime Minister of North Vietnam, was losing large numbers of soldiers to malaria. He petitioned China to help find a cure for it.
About 600 scientists, including military personnel, scientists and practitioners of Western and traditional Chinese medicine, were convened and divided into three teams: one for conducting clinical studies, one for looking into traditional Chinese medicine and the third for developing synthetic compounds. Among their goals was a cure for chloroquine-resistant malaria.
Would they succeed in finding a solution to one of humankind’s oldest and most resilient scourges? The story of Project 523 is a sharp reminder of how the wisdom of traditional forest medicine continues to light the way for modern medicine, even though many people still consider it the slightly suspect little brother of science.
By that time, 240,000 compounds had been unsuccessfully tested worldwide for their action against malaria. Some of the 380 or so plant extracts examined by Project 523 had shown promise, but inconsistently. Help arrived one day in the form of a book by the fourth-century physician Ge Hong, Zhouhou Beiji Fang (literally The Handbook of Prescriptions for Emergencies). In its pages, Dr Tu Youyou and her team stumbled upon a prescription for using the extract of quinghao (the sweet wormwood plant, Artemisia annua) to treat malarial fever. Following the recipe exactly, they obtained a purified extract that successfully cured malaria in laboratory mice and monkeys.
In 1971, Tu Youyou’s team isolated artemisinin, the active compound in sweet wormwood that was powerfully effective against malaria. Two years later, she synthesized dihydroartemisinin, a compound more soluble and potent than nature’s original. Since then, scientists have synthesized several artemisinin derivatives, notably artemether and artesunate.
Artemisinin is now WHO’s recommended first and second line of treatment for malaria. In 2015, Tu Youyou won the Nobel Prize in Physiology or Medicine for her ground-breaking work on malaria.
Artemisinin is neither the first nor the last example of how the healing bounty of the earth’s forests has been saving human lives for millennia — and continues to do so even in the age of computerized medicine, artificial intelligence and gene manipulation.
A timeless history of healing
There is ample evidence that the ancients had extensive knowledge of the healing powers of plants, including many of forest origin. The Chinese emperor Shen Nung created a compendium of medicinal plants and their uses in 2,800 BCE; Egyptian papyruses from 1,550 BCE list plant remedies, and there is a 7th-century BCE compilation of Assyrian therapeutic plants. Plants were described in detail in the Greek Historia Plantarum, written by Plato’s disciple Theophrastus in the 4th century BCE. Another Greek, the physician Dioscorides, authored De Materia Medica around 75 BCE. It remained the sole reference for medicinal plants for the next 1,500 years. The Indian Ayurvedic and the Arabic Unani systems of medicine include deep and ancient knowledge of the healing properties of plants, much of it orally transmitted before documentation became possible.
Recently, startling archaeological evidence emerged indicating that even Neanderthal humans may have known something of the medicinal value of forest plants.
A news item in February 2020 announced the 11th Neanderthal skeleton found at Shanidar cave, a burial site up on Kurdistan’s Bradost Mountain in northern Iraq, about 500 miles from Baghdad. They named it Shanidar Z and ascertained through carbon dating that it was around 70,000 years old, making it the oldest one found in the cave.
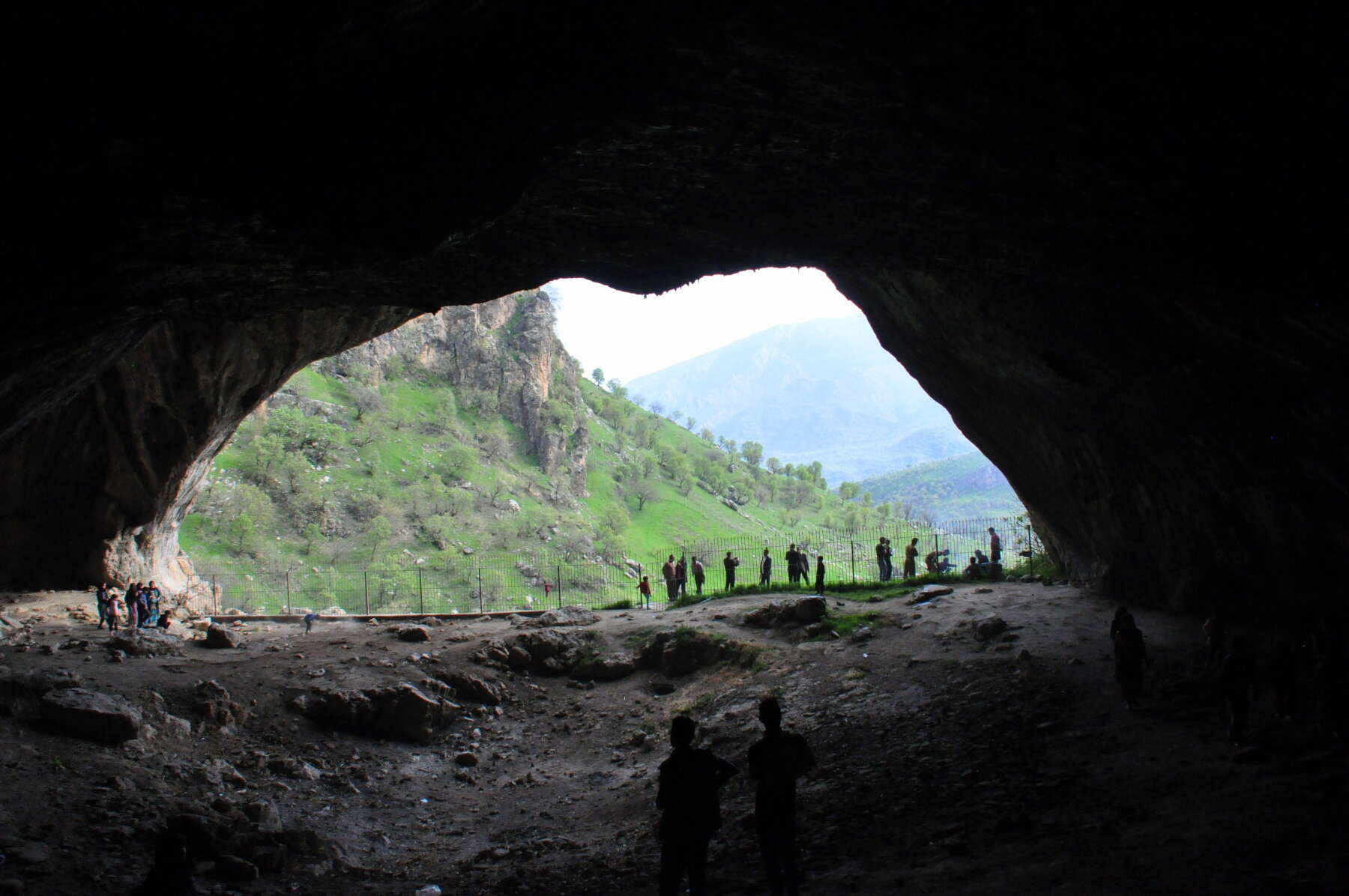
The similarities with Shanidar 4, the most complete Neanderthal skeleton also found there, in 1963, were striking. Both were middle-aged adults between 30 and 45 years old whose bodies had been carefully posed, indicating a formal, ritual burial. Most intriguing was the discovery that Shanidar 4 and Shanidar Z were ‘flower burials’ – clumps of pollen of plants from nearby forests and woodland meadows were arranged around the skeletons.
Dr. Ralph S. Solecki of Columbia and Dr. Arlette Leroi‐Gourhan, a French expert on identifying fossil pollen, concluded that Shanidar 4’s body had been laid upon the branches of a shrub known today to contain ephedrine, a nerve stimulant. Six of the seven species of wildflowers that had been placed around the body – including yarrow (Achillea millefolium), cornflower (Centaurea cyanus), St. Barnaby’s thistle (Centaurea solstitialis), ragwort (Senecio jacobaea), grape hyacinth (Muscari spp.), woody horsetail (Equisetum sylvaticum), and hollyhock (Alcea spp.) – are known to have medicinal properties. Two of them, the woody horsetail and hollyhock, grow only in forests and wooded areas. The Shanidar men remain the most compelling evidence that our earliest hominin ancestors may have understood the medicinal value of forest plants.
More evidence, equally compelling, came in 2012 from excavations at El Sidrón in Spain. Neanderthals lived here between 47300 and 50600 BP (Before Present, taken as 1950 for archaeological purposes) when this region enjoyed a mild climate and deciduous forests. Examining the dental plaque of one of the skeletons, researchers found traces of camomile and, as with the Shanidar remains, yarrow.
Yarrow, a flowering plant from the Asteraceae family common across temperate regions, has an extended history of medicinal use as an astringent. Bioactive compounds in chamomile (Matricaria chamomilla) are believed to have antimicrobial and anti-inflammatory properties and help alleviate general anxiety. In 2017, researchers discovered that the Sidrón skeleton also had a dental abscess and had been chewing poplar, which contains salicin, a precursor of aspirin.
Self-medication, or pharmacognosy, is common among many creatures, from caterpillars to apes, primarily for expelling parasites. The findings of El Sidrón and Shanidar may not indicate systematic study and knowledge of plant medicine among Neanderthals. Still, they suggest that forests have been integral to human health for far longer than recorded history.
Forests full of secrets
About 31% of the planet’s land area, or 4.06 billion hectares, is covered by forests, defined as “land spanning more than 0.5 hectares with trees higher than 5 meters and a canopy cover of more than 10%, or trees able to reach these thresholds in situ.” More than a third of this is primary forest, that is, naturally regenerated forest with native species and no sign of human encroachment or activity.
There are estimated to be 3.04 trillion trees on the planet, of which about 1.3 trillion are in tropical and subtropical forests and 740 billion in the boreal forests of northern Europe, Asia and North America. Scientists have identified 58,497 tree species so far, but an unknown number, perhaps in the tens of thousands, may be waiting to be counted.
Estimating the number of forest-growing medicinal plants is difficult, mainly because only a fraction have come under scientific scrutiny. The 2023 report of the World Flora Online (WFO), a 2020 initiative run by a consortium of over 50 botanical institutions, lists 377,749 plant species without segregating forest plants or evaluating their medicinal value. There are believed to be hundreds of thousands more species awaiting identification and classification.
“I am a scientist, not a poet. And I have been investigating the science behind that feeling for many years. I want to know why we feel so much better when we are in nature.”
Qing li, japanese society for forest medicine
Tropical forests cover roughly 12% of the Earth’s land surface but harbor half the planet’s biodiversity. A close look at the Amazon rainforest, the world’s largest at 2,7222,000 square miles, gives a sense of the epic richness of unexplored medicinal forest plants. Accounting for one-third of global plant biodiversity, the Amazon is home to about 90,000 plant species. Only a handful of them have undergone intensive screening for their therapeutic values.
WHO estimates that approximately 80% of the world’s population, representing 88% of the world’s countries, rely on herbal medicines, many of them from forest plants, to meet their primary healthcare needs. According to the Food and Agricultural Organization of the United Nations (FAO), over 50,000 plants may be in indigenous use for medicinal purposes worldwide. Over 40% of current medicinal drugs – including aspirin, artemisinin, quinine, paclitaxel, physostigmine and others – are derived from forest products. Half of all anti-cancer drugs introduced since the 1940s are either natural products or their derivatives.
Curiously, the pharmaceutical value of medicinal forest plants comes from certain organic compounds that have no direct connection with their growth and reproduction. Known as secondary metabolites, they contribute to the plants’ long-term health and appearance. According to conservative estimates, there are as many as 400,000 secondary plant metabolites on the planet, of which only about 10,000 have been chemically characterized. Some act as hormones, others add color and fragrance to the flowers and fruits, and yet others produce chemical defenses against pests. Of these, the most important for the development and production of drugs are alkaloids, ring-shaped bio compounds with at least one nitrogen molecule. Plants containing alkaloids have been shown to be bioactive against inflammation, tumors, and bacterial, fungal and viral infections. They have been deployed in medications, recreational drugs and entheogenic rituals.
About 27,000 alkaloids, 21,000 of them from plants, have been described so far, including well-known ones like nicotine, atropine and morphine; protoalkaloids like ephedrine, mescaline and tryptamine; and others such as caffeine, theobromine and theophylline, known as pseudo-alkaloids. Scientists estimate that tens of thousands more may be in the wings, waiting to be discovered. Today, the forests’ unexplored drugs are the holy grail of the pharmaceutical industry.
From forests to pharmacies
How does a medicinal forest plant known and used by a few traditional communities turn into a globally marketed, multi-million dollar pharmaceutical product?
Indigenous people used plants or their parts individually or as ingredients in herbal medicines to treat symptoms, having no concept of disease. It was not until Louis Pasteur proposed the germ theory in the 1860s that scientists began to look for invisible microbes that caused diseases, and specific chemical compounds that could fight them.
However, evaluating forest plants at random for new medicinal compounds – known as New Chemical Entities or NCEs – is time-consuming, costly and comes with a low success rate. At the turn of the century, only about 15% of the 250,000 or so identified species of higher plants had undergone phytochemical screening, and an even smaller number, around 6%, had been evaluated for their biological properties.
The modern strategy is based on dipping into the vast repertoires of traditional medicines and the ethnopharmacological knowledge of indigenous people to identify candidate drugs that could yield therapeutic NCEs. Natural compounds obtained in this way from botanical sources could include:
Biologically active compounds ready to be used directly as medicines. One of the best-known of these is quinine, found in the powdered bark of the cinchona tree, which was widely used by indigenous South American people for treating fevers. Another example is artemisinin, derived from the sweet wormwood plant (Artemisia annua) and used directly to treat malaria.
Lead compounds with specific biological activity that could be chemically tweaked to yield more robust and potent versions. A lead compound is a specific chemical compound, usually from an active ingredient or plant extract, that shows biological or pharmacological promise in early trials. It can be developed into a therapeutic drug by being modified and optimized to make it more efficient, less toxic, more easily absorbed, excreted and so on. Some examples of this are:
- Digoxin, extracted from the foxglove plant (Digitalis purpurea), which spurred the development of similar cardiac glycosides also for treating heart conditions.
- Salicin, from the bark of the willow tree (Salix spp.), and salicylic acid, extracted from meadowsweet (Filipendula ulmaria), which were precursors of aspirin, the world’s single most prescribed medicine today. The body converts salicin to salicylic acid, a compound with anti-inflammatory properties but unpleasant gastrointestinal side effects. Although aspirin’s molecular template came from salicylic acid, it is a purely synthetic chemical with remarkable anti-inflammatory, antipyretic and analgesic properties but without the side effects of salicylic acid.
- Taxol, known also as Paclitaxel, derived from the Pacific yew tree (Taxus brevifolia), which inspired the semi-synthetic drug analog docetaxel, used against breast, lung, prostate and stomach cancers.
- Podophyllotoxin, extracted from the May apple plant (Podophyllum peltatum), whose semi-synthetic derivatives like etoposide and teniposide are used to treat cancers.
- Procaine, synthesized from a structural template based on cocaine, which is the active ingredient of the coca bush (Erythroxylum coca Lam.).
Chemical templates, called pharmacophores, whose structures can yield entire families of drugs for treating a specific condition. Unlike a lead compound, a pharmacaphore is an abstract framework that identifies the must-have molecular features that make a compound effective against a particular biological target like a tumor. In forest medicine, a pharmacophore describes the parts of a natural molecule extracted from a forest plant that are critical for its medicinal effects. Apharmacophore can serve as the basis for similar compounds in other plants and for synthesizing new drugs.
- An example of a pharmacophore is scopolamine, also known as hyoscine or Devil’s Breath, a tropane alkaloid and anticholinergic drug extracted from plants such as henbane (Hyoscyamus niger), jimson weed (Datura stramonium), deadly nightshade (Atropa belladonna) and mandrake (Mandragora officinarum). It is popularly used to treat motion sickness and nausea.
- Once scientists identified the pharmacophore, or the molecules critical to scopolamine’s therapeutic effects, they were able to create several other drugs with similar biological action, including Darifenacin (Enablex) and Tolterodine (Detrol) for treating overactive bladders; Trihexyphenidyl (Artane) and Benztropine (Cogentin) for treating Parkinson’s disease and other movement disorders; and cognitive enhancers like Donepezil (Aricept) and Rivastigmine (Exelon), used in treating Alzheimer’s disease.
Many of the most widely used drugs today would not have come to light if not for their use by traditional communities in ethnomedicine. Some of the most riveting examples of the progression from forest plant to pharmaceutical drug are physostigmine; curare; quinine; vinblastine and vincristine; cortisone; and calanolide A.
Physostigmine: The Case of the Judicial Bean
In 1846, an English physician and botanist, William Freeman Daniell, after spending a year in the Old Calabar, at the delta of what is today’s Niger River in Nigeria, described a curious custom locally known as “chopping nut”, used to determine guilt when an accused person was brought before the kings and chiefs of a tribal court. The beans of a poisonous local plant called eséré would be pounded in a mortar and soaked in water until it turned milky. The accused would be made to drink this and walk about until the poison kicked in. Death was considered proof of guilt. If he survived the ordeal, he would be set free, judged innocent.
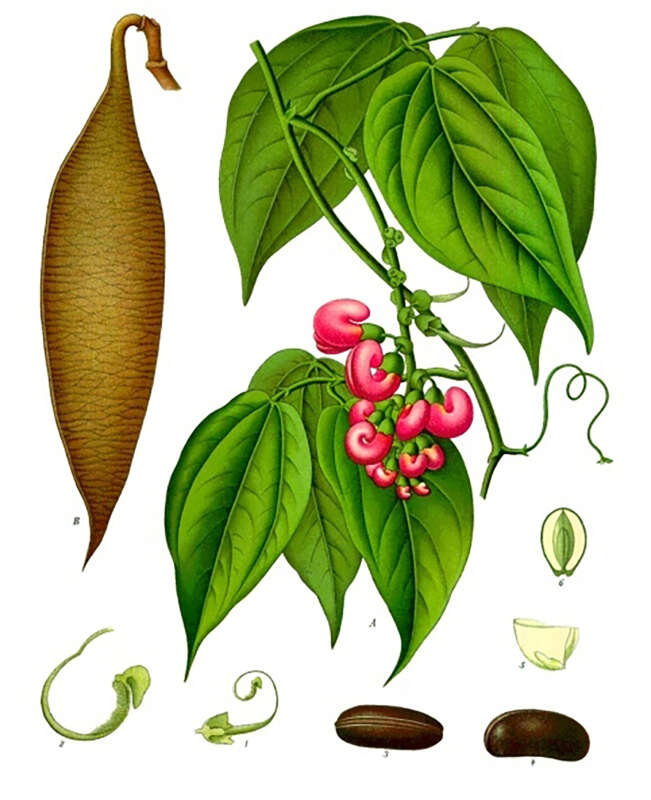
In the mid-19th century, some samples of the plant, known as the Calabar bean (Physostigma venenosum) reached England. In 1846, its active component, physostigmine, was identified by toxicologist Sir Robert Christison, who first tried out the bean on rabbits before one day swallowing a quarter of a bean on an empty stomach. Experiencing a series of alarming symptoms, including auricular fibrillation, torpidity and spasms, he saved himself by drinking his soapy shaving water to induce vomiting. On completing his research, he suggested in his paper that death by Calabar bean might be a more humane alternative to hanging.
It was not until 1855 that Thomas Richard Fraser, a Scottish pharmacologist, isolated and concentrated the active alkaloid in the Calabar bean, physostigmine, also known as eserine. In an award-winning paper, he wrote that physostigmine increases the salivary, gastric, and bronchial secretions, boosts intestinal tone and stimulates motility, slows the heart, dilates the peripheral blood vessels and constricts the eye’s pupils.
Physostigmine was synthesized 80 years later by Percy Julian, an African-American chemist and research fellow at DePauw University, Indiana. Available as Antilirium and Isopto Eserine, as well as eserine salicylate and eserine sulfate, one of physostigmine’s first uses was to treat glaucoma, based on its ability to cause miosis, or constriction of the pupils. Physostigmine derivatives have also been effective against Alzheimer’s disease and myasthenia gravis, characterized by muscular weakness caused by a communication breakdown between nerves and muscles.
Curare: From Poison Arrow to Surgical Knife
While exploring the jungles of Guyana, Sir Walter Raleigh noticed tribal hunters blowing poison-tipped darts through bamboo blowpipes to kill prey. The potent poison, which he named curare, could kill a bird in about two minutes, while small mammals took up to 10 minutes. A larger animal like a tapir might survive for about 20 minutes.
Curare was most frequently made with young scrapings of the bark of Strychnos toxifera or S. guianensis, and of the Menispermaceae family, especially Chondrodendron tomentosum or Sciadotenia toxifera. These would be boiled for about two days, sometimes with snake venom or venomous ants added, to obtain a dark, heavy, viscid, and bitter paste. Its potency would be tested by counting the number of leaps a frog would take after being pricked.
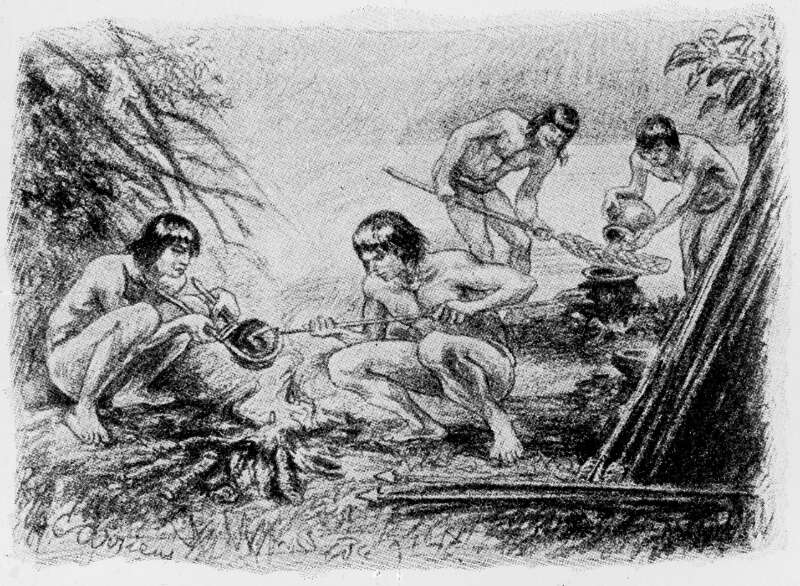
Credit: Wellcome Library, London
In 1825, samples of curare reached Europe, and a series of discoveries about its action quickly followed. Its muscle-relaxing property comes from the alkaloids curarine and tubocurarine, and in 1850, George Harley showed that it could treat tetanus and strychnine poisoning. By 1887, it was being marketed for medical use by Burroughs Welcome. In 1912, Arthur Lawen pioneered the use of curare during surgery, though his findings did not receive widespread attention because they were published in German. In 1940, Abram Elting Bennett used curare to reduce trauma during convulsive therapy for spastic disorders in children.
In 1942, Harold Griffith, a Canadian doctor working as Chief of Anesthesia at the Homeopathic Hospital in Montréal, Quebec, made one of the most consequential medical advances of the last 100 years when he used curare as a muscle relaxant to augment general anesthesia during an appendectomy. This ushered in an era when using muscle relaxants during anesthesia became standard practice, profoundly transforming the safety and effectiveness of anesthesia. Today it is difficult to imagine how surgical operations we take for granted, such as open-heart surgery, organ transplant, and brain, thoracic, and abdominal surgery, could have developed without curare.
Quinine: The Bark That Created Empires
“What the story of quinine shows is that biodiversity and human health go hand in hand,” says Patricia Schlagenhauf, a professor of travel medicine at the University of Zurich. “People often think of plant-based remedies as ‘alternative medicine’, but it is to plants that we owe some of the major medicinal breakthroughs in human history.”
Though there is considerable debate about whether malaria even existed in South America before the Spanish conquerors arrived, there is no doubt that the Quechua people of Peru, Bolivia and Ecuador knew that the bitter bark of the cinchona tree, ingested in powdered form with water, was superbly effective against fever and shaking chills.
Spanish Jesuits brought the cinchona bark to Europe in the late 1620s, where Protestants promptly labeled it a “Jesuit powder” and refused to have anything to do with it. Word got around that it was a superior cure for fevers and chills, though because of its bitter taste, it was best drunk mixed with some fine wine. Still, the absence of guidelines regarding quantity and dosage prevented total acceptance of cinchona as a reliable medicine.
All that changed in 1820, when the French scientists Pierre Pelletier and Joseph Caventou extracted quinine from the cinchona bark – and just in time too, because Europe’s colonizers were getting down to serious empire-building in the hot, tropical countries of the east. Procuring enough quinine for treating soldiers with malaria during their colonial campaigns became a key point of military strategy. For them, the discovery of quinine came not a moment too soon.
As demand for quinine skyrocketed, the newly independent countries of Peru, Ecuador, Colombia and Bolivia realized they were sitting on a gold mine and imposed high tariffs and strict controls on the export of seeds and plants. The British countered by starting their own cinchona plantations in India, albeit without much success. The Dutch fared better in Java with the commercial production of Cinchona ledgeriana, a species with a high quinine content. By the 1880s, they were the undisputed kings of the global quinine trade.
When the Japanese drove the Dutch out of Java during World War II, they took over its cinchona plantations, creating a new era of quinine shortage and increasing the pressure on pharmaceutical companies to synthesize anti-malarial compounds based on their lessons from studying quinine. Chloroquine was among the most popular of these and remained the gold standard of malaria prophylaxis and treatment until mosquitoes developed resistance to it.
Overharvesting has made the cinchona tree an endangered species in South America today. Explorers in the Ecuadorian Andes counted about 25,000 cinchona trees in 1805. A century or so later, in the same forest, now part of the Podocarpus National Park, you can see no more than 29 trees.
Vinblastine and Vincristine: From Living Rooms to Cancer Wards
Its leaves, flowers, roots and other parts have been used traditionally to treat a bewildering range of health conditions, including the symptoms of diabetes, dengue fever, dysentery, insect bites, skin wounds and infections, toothache and sore throat. In Madagascar, its bitter, astringent leaves are used as an emetic, while its roots are known to be purgative, vermifuge, depurative, hemostatic and a toothache remedy. The juice of its leaves is used to treat wasp stings in the Indian states of Odisha and Assam. Its floral extracts are considered safe as eyewash for infants in Cuba and Jamaica, and used to treat asthma in the Bahamas, hypertension in Bermuda, dysmenorrhea in Indo-China, stomach ulcers in Uganda and mature abscesses in Botswana. In China, its formidable powers have long been applied in treating leukemia, lymphosarcoma and giant follicular lymphoma.
This Swiss army knife of a plant is the pretty Madagascar periwinkle (Catharanthus roseus) of the family Apocynaceae. Once listed under the genus Vinca as Vinca rosea, it is known to the Malagasy people as vonenina or ‘the flower of remorse’. Its vibrant pink flowers and inherent hardiness make it an ornamental favorite in living rooms, but in the annals of forest medicine, this plant is famous for its potent cancer-fighting powers.
Scientists looking into the healing powers of the Madagascar periwinkle in the early 1950s found that every part of the plant was rich with terpenoid indole alkaloids (TIAs), some with distinct medicinal properties. More than 130 TIAs were extracted, and in vitro and animal studies hinted at the potential for anti-diabetic properties. However, the two alkaloids that stood out had nothing to do with diabetes: vincristine (VCR) and vinblastine (VBL) seemed powerful against certain cancers.
Vincristine is the only anti-leukemic drug that drastically reduces white blood cell count. Since it was introduced in the 1950s, it has dramatically boosted the survival rate of children with leukemia from 20% to 80%. Vinblastine, similarly, has proven its strength in fighting Hodgkin lymphoma, a group of blood cancers that start in the white blood cells and affect the lymphatic system.
Unfortunately, VBL and VCR exist only in minuscule amounts in the aerial parts of the plant, and their synthetic versions such as vinorelbine and vindesine are notably less effective. For example, fully synthetic vincristine is only one-fifth as effective as the natural product derived directly from the plant. A kilogram of vinblastine is estimated to cost $2 million, and vincristine is $15 million. The global vinca alkaloid market in 2022 was $85 million and is expected to touch $156.32 million by 2031. With rising global demand for VBL and VCR, extensive research is going on to deploy cell-tissue culture and mutation induction as alternative production methods, in addition to other proven techniques.
The pharmaceutical exploitation of the Madagascar periwinkle has also cast a light on biopiracy, or the use of biological materials and traditional knowledge without fair compensation or acknowledgment to the indigenous peoples who have been custodians of the knowledge and medical practices for generations. There is growing pressure for more equitable benefit-sharing arrangements under the framework of the Convention on Biological Diversity (CBD). This agreement, ratified by 196 countries, aims to ensure the fair and equitable sharing of benefits arising from the use of genetic resources. Sadly, in the case of VBL and VCR, not much has changed for the indigenous people of Madagascar.
Cortisone: The Elephant’s Foot in the Room
Why would scientists turn to forests to produce a chemical that the human body manufactures naturally in the adrenal glands? A driving factor was the surge in demand after a scientist called Philip Hench showed, in 1948, that a certain Compound E dramatically relieved the pain of patients with rheumatoid arthritis. Patients who had been disabled for years were trying to dance. One insisted on taking several baths the same day to compensate for lost time.
Compound E was one of several unidentified hormones that Hench’s friend at Mayo Clinic, Edward Kendall, had separated and characterized back in 1929. Compound E, it turned out, was cortisol, the body’s natural response to stress and inflammation, created in the liver by the action of an enzyme on an inert chemical called cortisone. Injected directly into painful joints and inflamed areas, it provided dramatically effective relief. In 1948, the pharmaceutical giant Merck Sharp & Dohme began synthesizing cortisone from diosgenin, a compound extracted from Mexican wild yams. With it began a global gold rush for other forest plants from which diosgenin could be extracted.
The story of cortisone illustrates the conflict between corporate interests and the conservation of natural treasures and how indigenous people can ensure that they get a fair share of the benefits of forest plants.
In the early 1950s, Boots, a prominent British pharmaceutical company with a factory in Nottingham, became interested in getting on the cortisone bandwagon and began looking for forest sources that would not be as expensive as Mexican wild yam. Their attention fell on the unprepossessing South African wild yam (Discorea sylvatica), also called Elephant’s Foot based on its shape, which traditional healers had been using for generations because of its healing properties.
Through a subsidiary called Biochemico, Boots began producing diosgenin made from Discorea sylvatica, processing about 100 tons per month. A report by Biochemico speaks of hiring locals to dig up 6,000 tons of wild yams from the eastern and northeastern parts of the country. It was clear to provincial conservation officials that if left unchallenged, the pharmaceutical giant would harvest wild yams to their extinction.
The Natal Parks Board began enforcing countermeasures, worried that one of their natural treasures was being pirated out of existence. By 1960, they succeeded in revoking Boots’ permits, forcing the company to stop diosgenin production from South African wild yams. However, by then, local healers had realized that not only were they the custodians of traditional knowledge but also its guardians, responsible for preventing the exploitation of forest plants for profit without any benefit to them or their communities. The South African San Council and National Khoisan Council, representing indigenous people, would build benefit-sharing into future ethnobotanical partnerships with corporations and institutions.
Calanolide A: It Takes a Forest to Fight HIV
1987 was an eventful year for AIDS. The Emmy-award-winning pianist Liberace died, and an autopsy revealed an AIDS-related illness to be the cause of death. The US Food and Drugs Administration approved zidovudine, better known as AZT, the first-ever medication against AIDS. Princess Diana made world headlines when she was photographed shaking hands with an AIDS patient. In six years, AIDS has become the single most talked-about and researched disease on the planet.
That year, in a swamp forest at Lundu, Sarawak, Malaysia, a botanist called John Burley had been busy collecting a kilogram of fruits, leaves and twigs from each type of plant they saw. He and his team were a part of one of the world’s largest anti-HIV screening programs, conducted by the National Cancer Institute to find new substances for treating HIV/AIDS and cancer. Between 1987 and 1996, they screened over 30,000 plant extracts.
One of the samples he sent for screening, labeled ‘Burley-and-Lee-351’, showed no action against cancer cells, but when tested against HIV-1, it stopped the virus from replicating. It came from a tree known locally as Bintangor (Calophyllum lanigerum var. austrocoriaceum).
There was a small problem. Calophyllum trees were scarce and difficult to find. More importantly, only 0.05% of the twigs and leaves consisted of Calanolide A. It was only in 1996 that scientists successfully synthesized Calanolide A in the laboratory. They also discovered a sister compound, which they named Calanolide B, in the latex of a related species, Calophyllum teysmannii var. Innophylloide.Though it was less potent than Calanolide A, its yield from raw materials was much higher.
A rare forest plant had shown its potential to fight HIV1. Scientists had run with the ball and created a spectrum of powerful anti-HIV drugs. Many other plants of the Calophyllum species biosynthesize Calanolide A, including Calophyllum brasiliense, Calophyllum inophyllum, Calophyllum teysmannii, and Clausena excavata. Other similar Calanolides have also been found, expanding the range to Calanolide F.
Some other outstanding examples of widely used, lifesaving modern medicines with their origins in forests are digoxin from the foxglove, Digitalis purpura, used to treat cardiac conditions; reserpine from the Indian snakeroot, Rauwolfia serpentine, effective in managing hypertension and controlling severe agitation in patients with mental health problems; paclitaxel from the Pacific yew, Taxus brevifolia; hypoglycemic agents such as metformin from the French lilac, Galega officinalis; and antibiotics such as penicillin from the fungus Penicillium notatum.
Over the last decade or so, the growing pressure from emerging infectious diseases, the rise of antimicrobial resistance and the urgent need for better therapeutic drugs and treatments have put pharmaceutical companies in a bind. Combing through thousands of forest plants in the hope of finding new medicines with no guarantees of a breakthrough is unviable and time-consuming, and the pharmaceutical industry has begun shifting its focus to synthetic drugs instead, even while exploring more efficient strategies for identifying candidate drugs from ethnomedicine.
Paradoxically, popular interest in plant-based medicines, perceived as safer, more reliable and with fewer side effects, has been growing in recent years. However, ethical issues such as sustainability, preserving biodiversity, the ecological impact of scaling up and overharvesting, and equitable benefit-sharing with traditional forest communities have introduced layers of complexity to globalizing the forests’ medicinal treasures.
The Future is in the Mind
In this new, evolving environment, an area receiving intense attention is the psychotherapeutic potential of forest plants. Mental health accounts for 14% of the global disease burden, with almost three-quarters of the cases from low-income countries. In developed countries, mental health problems such as depression, anxiety disorders, post-traumatic stress disorder (PTSD) and alcohol and drug abuse are on the rise. Wars, mass migration, climate change, loss of habitat and livelihoods and other modern stresses exacerbate the problems. Mental health is on the edge of becoming one of the critical medical issues of our future.
Indigenous communities have long recognized that the forest not only heals the body but can also repair and build the broken mind. Psychoactive products from plants, fungi, cacti and mushrooms, such as mescaline, psilocybin, cannabis, opium and others, have been part of ethnomedicine for centuries. Originally dismissed as ‘hippie’ or New Age fads, some of them have begun commanding new respect as scientific research reveals their potential to heal, refresh and repair the mind.
Ibogaine is a powerful example of this.
Archaeological evidence from traces in charcoal indicates that the iboga plant (Tabernanthe iboga) was used 2000 years ago by indigenous West African people. Even today, forest-dwelling Punu and Mitsogo people in Gabon practice a spiritual discipline called Bwiti, whose rituals include the mind-altering root bark of the iboga plant, known to produce a dream-like state that promotes introspection and self-evaluation. The iboga root, consumed as a tea or in the form of scrapings, is at the heart of many Bwiti ceremonies, which begin at night and are marked by repetitive music, purification rites, vomiting and fasting. Everyone wears raffia skirts and ornamental shells or beads.
The scene might have been dismissed as a Hollywood depiction of mysterious Africa. That began to change in the 1960s, when heroin addicts began reporting that they had cured themselves of their addiction using ibogaine. More lab evidence emerged in 2017 when 30 people with opioid addiction were given 12 mg of ibogaine per kg of body weight for a year. Half of them had kicked their habit in a month without any painful withdrawal symptoms. A year later, seven participants remained addiction-free.
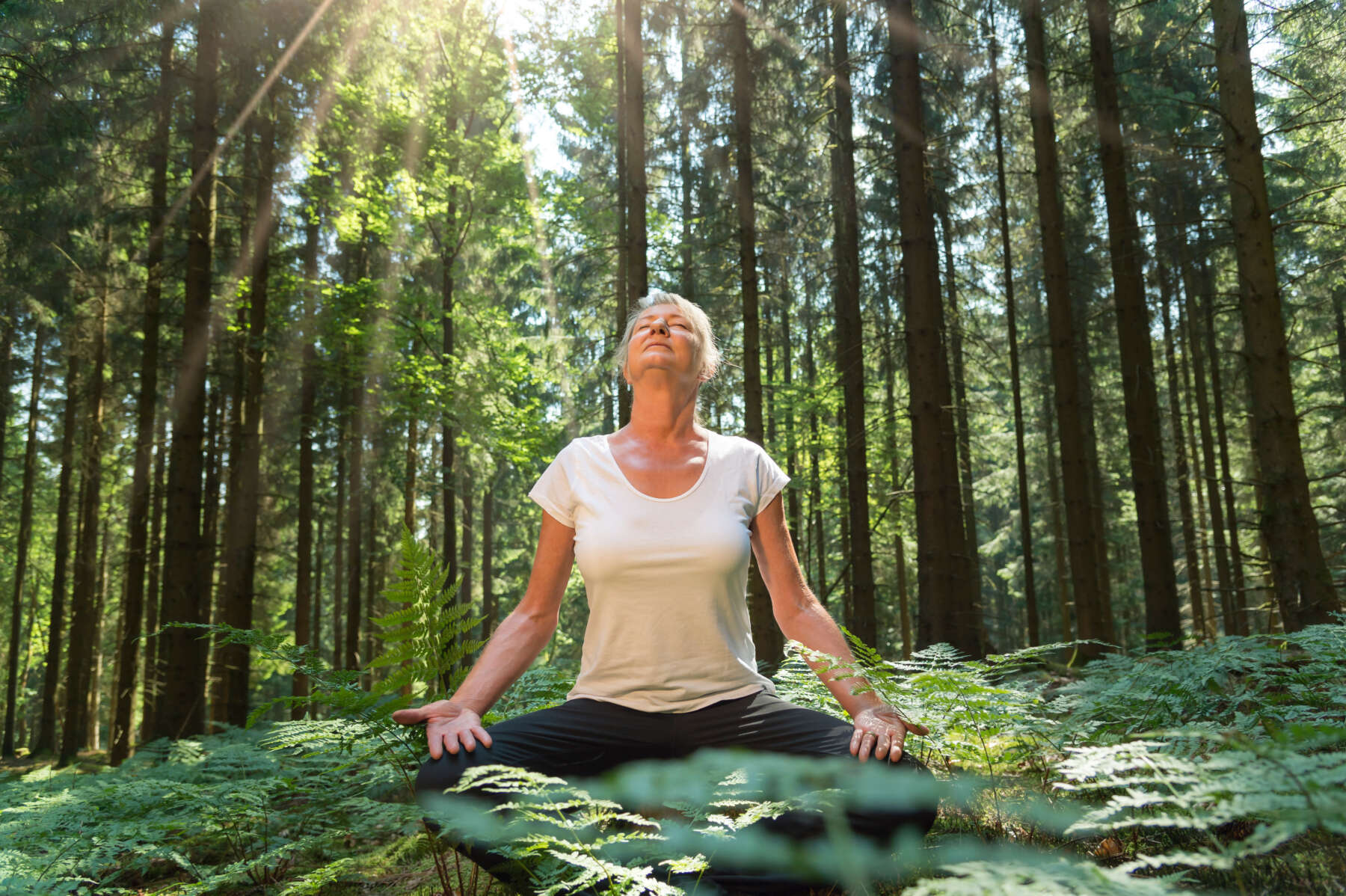
Ibogaine’s potential received a dramatic boost in January this year when Stanford University researchers working with Veterans Exploring Treatment Solutions (VETS) reported their findings on an experiment to test ibogaine’s effect on veterans suffering from traumatic brain injuries. TBI is the world’s leading cause of injury-related disability and also the hallmark injury of US veterans returning from recent military conflicts. Exposure to blasts from improvised explosive devices, roadside bombs, rockets and mortar shells, land mines, aerial bombs or rocket-propelled grenades is the most common cause.
The results were nothing short of stunning. The 30 or so participating veterans showed an average 88% reduction in their TBI symptoms and 81% in anxiety symptoms. They displayed improvements in concentration, information processing, memory and impulse control. Starting with an average disability rating of 30.2 (mild to moderate disability) on the disability assessment scale, they stood at 5.1 – no disability whatsoever – a month after treatment.
Scientists are still trying to understand how ibogaine achieves its effects but animal studies suggest that the ibogaine alkaloid, together with its metabolite, noribogaine, binds to nicotinic and opioid receptors, as well as dopamine and serotonin transporters.
Research is uncovering a cornucopia of forest plants that indigenous communities have been using for their psychotropic properties. A recent study in just one city, Goma in the Democratic Republic of Congo, unearthed 30 plant species, represented amongst 29 genera and 19 families, that were in use for treating anxiety, psychosis and depression. Of these, the desert candle (Euphorbia abyssinica) and the stinkweed (Cleome gynandra) were most commonly prescribed for anxiety, while the fleabane (Conyza sumatrensis) and the tassel flower (Emilia coccinea) were preferred for depression.
New, well-documented studies are pointing to an intriguing new potential: just regular contact with forests and green areas can contribute to human health, measured through levels of stress hormones, self-assessment scales and performance on standardized tests.
Walking through a forest increases positive emotions and reduces depression, fatigue, general anxiety, uncertainty and tension. Studies from the Republic of Korea showed that depressive patients scored lower on the Beck Depression Inventory after taking part in a forest healing program, while patients with alcoholism scored higher on a self-esteem measure.
Perhaps the most fascinating new window into the healing power of forests comes from the Japanese practice of shinrinyoku or forest bathing, which is nothing more than a leisurely walk in the forest. It started with a simple question asked by a Japanese student, Qing Li, in 1988: Why does a walk in the forest restore my vitality and make me feel so good? Today, as Chairman of the Japanese Society for Forest Medicine, he says, “I am a scientist, not a poet. And I have been investigating the science behind that feeling for many years. I want to know why we feel so much better when we are in nature.”
The answer, his research showed, lay in phytoncides, aromatic natural oils that plants and trees release. Containing volatile antimicrobial and insecticidal compounds, phytoncides create a protective shield around forest trees and plants to protect them from bacteria, fungi and insect predators. Inhaled by humans, they bring a bounty of benefits: they reduce sympathetic nervous activity while increasing parasympathetic nervous activity and regulate the balance of autonomic nerves, thus lowering blood pressure and heart rate. Phytoncides also suppress the sympathetic ‘fight or flight’ system and boost the parasympathetic ‘rest and recover’ system. Participants reported a sense of well-being, slept better and longer, and enjoyed these effects for weeks.
Some studies indicated that forest bathing indirectly influences the endocrine and immune systems, lowering urinary adrenaline and boosting the activity of natural killer cells, which attack infected cells and cancerous tumors. One study Li conducted with the Nippon Medical School featuring 12 healthy participants showed that the boosted natural killer cell activity they experienced after a weekend in the forest lasted a month.
Among the observed effects were:
- Improved immune response: A 2-hour walk in the forest increases natural killer cell activity that can last for days.
- Anti-inflammatory activity: Terpenes released by the forest fight inflammation and reduce oxidative stress.
- Enhanced mood: Exposure to forest air reduces blood cortisol levels, while the phytoncide β-pinene shows anti-depressive properties.
- Improved sleep: Phytoncides like α-pinene give you a better night’s sleep.
- Lower blood glucose: Inhaling the compounds in forest air helped reduce blood glucose levels.
The term ‘forest bathing’ was coined by Tomohide Akiyama, the Head of the Japanese Forestry Agency, in 1982, and the practice has steadily grown in Japan. Between 2004 and 2012, the Japanese government spent $4 million on a nationwide research project to investigate how forests, including individual aspects such as the odor of wood, the sound of running streams and forest scenery, affect human mental and physical health. Some findings demonstrated improvements in blood pressure, heart rate, stress hormone levels and autonomic nervous activity.
The Forest Therapy Society started registering certified forest therapy bases (FTBs) and forest therapy roads (FTRs) in 2006 as scientifically approved locations in forests and urban environments that enhance health and encourage relaxation. Forest therapy roads are gently sloping paths wider than regular sidewalks, designed for a 20-minute walk. Japan now has 62 designated therapeutic woods, attracting about 5 million visitors annually.
In a world where forests are disappearing and thousands of species are going extinct every day due to climate change and human activity, it may seem contrary to say that humans are deeply drawn to forests. Yet this is the implicit belief of biologist Edward O. Wilson, winner of the 2007 TED prize given to individuals who have “shown that they can, in some way, positively impact life on this planet”. Humans, he says, have a fundamental urge to merge with nature, though it may be impossible to measure scientifically.
Perhaps he knows something the world has forgotten — that we need forests to survive as a species. In 1984, Roger Ulrich, a medical researcher, published a study that analyzed a decade’s worth of records from a Philadelphia hospital to prove that patients whose windows opened to views of trees and greenery recovered sooner and were less depressed than those forced to stare at buildings.
A forest is a healing force. Even when it stands at a distance.
Click Here for References
Hsu, E. (2006). Reflections on the ‘discovery’ of the antimalarial qinghao. British Journal of Clinical Pharmacology, 61(6), 666-670. https://doi.org/10.1111/j.1365-2125.2006.02673.x
Traditional medicine has a long history of contributing to conventional medicine and continues to hold promise. WHO Newsroom. 10 August 2023. https://www.who.int/news-room/feature-stories/detail/traditional-medicine-has-a-long-history-of-contributing-to-conventional-medicine-and-continues-to-hold-promise
FAO. 2020. Forests for human health and well-being – Strengthening the forest–health–nutrition nexus. Forestry Working Paper No. 18. Rome. https://doi.org/10.4060/cb1468en
WHO Global Traditional Medicine Centre. https://www.who.int/initiatives/who-global-traditional-medicine-centre
Crowther, T., Glick, H., Covey, K. et al. Mapping tree density at a global scale. Nature 525, 201–205 (2015). https://doi.org/10.1038/nature14967
Buckley, S., Hardy, K., & Huffman, M. (2013). Neanderthal self-medication in context. Antiquity, 87, 873-878. https://doi.org/10.1017/S0003598X00049528
Leroi-Gourhan, A., “The flowers found with Shanidar IV, a Neanderthal burial in Iraq.”, Science, vol. 190, pp. 562-64, 1975 http://dx.doi.org/10.1126/science.190.4214.562
Marripati, D. (2023). Plant alkaloids: An overview. Journal of Plant Biochemistry and Physiology, 11, 262.
Pereira, A. G. et al (2023). Plant alkaloids: Production, extraction, and potential therapeutic properties. In M. Carocho, S. A. Heleno, & L. Barros (Eds.), Natural secondary metabolites: From nature, through science, to industry (pp. 157–200). Springer International Publishing.
Antonelli, A. et al (2023). State of the World’s Plants and Fungi 2023. Royal Botanic Gardens, Kew. DOI: https://doi.org/10.34885/wnwn-6s63
Firn, R. D., & Jones, C. G. (2003). Natural products – A simple model to explain chemical diversity. Natural Product Reports, 20, 382-391.
Wink, M. (2003). Evolution of secondary metabolites from an ecological and molecular phylogenetic perspective. Phytochemistry, 64, 3-19.
Fabricant, D.S.; Farnsworth, N.R. The value of plants used in traditional medicine for drug discovery. Environ. Health Perspect. 2001, 109 (Suppl. 1), 69–75.
Jex, C. (2015). There are now 3.04 trillion trees on earth. September 2015, ScienceNordic.
2023 Report of World Flora Online https://www.worldfloraonline.org/
Paleomedicine and the use of plant secondary compounds in the Paleolithic and Early Neolithic
https://www.researchgate.net/publication/331155660_Paleomedicine_and_the_use_of_plant_secondary_compounds_in_the_Paleolithic_and_Early_Neolithic
Hardy K. Paleomedicine and the use of plant secondary compounds in the Paleolithic and Early Neolithic. Evolutionary Anthropology. 2019;28:60–71. https:// doi.org/10.1002/evan.21763
Healthy forests, healthy planet, healthy humans. United Nations Department of Economic and Social Affairs. March 2023. https://www.un.org/en/desa/healthy-forests-healthy-planet-healthy-humans
Mans, D. R. A. (2013). From forest to pharmacy: plant-based traditional medicines as sources for novel therapeutic compounds. Academia Journal of Medicinal Plants, 1(6), 101-110. Retrieved from http://www.academiapublishing.org/ajmp
Cragg, G. M., Newman, D. J., & Weiss, R. B. (1997). Coral reefs, forests, and thermal vents: The worldwide exploration of nature for novel antitumor agents. Seminars in Oncology, 24, 156-163.
Global Forest Resources Assessment 2020 In brief – key findings (PDF). Rome: FAO. 2020. pp. 2–6. doi:10.4060/ca8753en. ISBN 978-92-5-132581-0. S2CID 130116768
Najmi, A., Javed, S.A., Al Bratty, M., & Alhazmi, H.A. (2022). Modern approaches in the discovery and development of plant-based natural products and their analogues as potential therapeutic agents. Molecules, 27(2), 349. https://doi.org/10.3390/molecules27020349
Atanasov, A. G. et al. (2015). Discovery and resupply of pharmacologically active plant-derived natural products: A review. Biotechnology Advances, 33(8), 1582–1614. Elsevier Inc.
https://pubs.acs.org/doi/pdf/10.1021/ba-1971-0108.ch001
https://www.jstor.org/stable/41109546?read-now=1&seq=1#page_scan_tab_contents
https://www.pbs.org/wgbh/pages/frontline/shows/heroin/etc/history.html
Scheindlin, S. (2010). Episodes in the story of physostigmine. Molecular Interventions, 10(1), 4-10. https://doi.org/10.1124/mi.10.1.1
https://www.bbc.com/travel/article/20200527-the-tree-that-changed-the-world-map
Caldas de Castro, M., & Singer, B. H. (2005). Was malaria present in the Amazon before the European conquest? Journal of Archaeological Science, 32(3), 337-340.
Miller, L. H., Rojas-Jaimes, J., Low, L. M., & Corbellini, G. (2022). What historical records teach us about the discovery of quinine. American Journal of Tropical Medicine and Hygiene, 108(1), 7-11. https://doi.org/10.4269/ajtmh.22-0404
The Tree That Changed The World Map. Vittoria Traverso, May 2020, BBC Travel.
Nejat, N., Valdiani, A., Cahill, D., Tan, Y. H., Maziah, M., & Abiri, R. (2015). Ornamental exterior versus therapeutic interior of Madagascar periwinkle (Catharanthus roseus): The two faces of a versatile herb. Scientific World Journal, 2015, Article 982412. https://doi.org/10.1155/2015/982412
Alam, P., & Sharafeldin, M. A. (2016). Limited production of plant-derived anticancer drugs vinblastine and vincristine. Planta Medica, 82(05). https://doi.org/10.1055/s-0036-1578706
Beinart, W., & Beinart, R. (2019). From Elephant’s Foot… to Cortisone’: Boots Pure Drug Company and Dioscorea Sylvatica in South Africa, c. 1950–1963. South African Historical Journal, 71(4), 644-675. https://doi.org/10.1080/02582473.2019.1668837
https://www.buchulife.com/blog/artlcle/south-african-san-council
Glyn, J. H. (1998). The discovery of cortisone: A personal memory. BMJ, 317(7161), 822A. https://doi.org/10.1136/bmj.317.7161.822a
https://worldrainforests.com/10anti_HIV.htm
https://skeptoid.com/blog/2014/05/22/the-mythology-of-asprin/
Szczeklik, A. (2006). The history of aspirin: The discoveries that changed contemporary medicine. Paths of Discovery (Pontifical Academy of Sciences, Acta 18). Vatican City. Retrieved from http://www.pas.va/content/dam/accademia/pdf/acta18/acta18-szczeklik.pdf
https://en.wikipedia.org/wiki/History_of_aspirin
Justin. Salicylic Acid: The Journey from Humble Willow to Aspirin. Nature’s Poisons. https://naturespoisons.com/2014/07/24/salicylic-acid-the-journey-from-humble-willow-to-aspirin/
Mash, D. C., Duque, L., Page, B., & Allen-Ferdinand, K. (2018). Ibogaine detoxification transitions opioid and cocaine abusers between dependence and abstinence: Clinical observations and treatment outcomes. Frontiers in Pharmacology, 9, 529. https://doi.org/10.3389/fphar.2018.00529
Brown, T. K., & Alper, K. (2018). Treatment of opioid use disorder with ibogaine: Detoxification and drug use outcomes. The American Journal of Drug and Alcohol Abuse, 44(1), 24-36. https://doi.org/10.1080/00952990.2017.1320802
Cherian, K.N., Keynan, J.N., Anker, L. et al. Magnesium–ibogaine therapy in veterans with traumatic brain injuries. Nat Med 30, 373–381 (2024). https://doi.org/10.1038/s41591-023-02705-w
Kyolo, S., Katuura, E., Bbosa, G., Mwebaza, N., Kibendelwa, Z. and Nakasujja, N. (2022) Medicinal Plants Used in Management of Various Mental Illnesses in Goma City, Democratic Republic of Congo. Neuroscience and Medicine, 13, 17-42. doi: 10.4236/nm.2022.131002
Ayahuasca and its Potential to Treat PTSD. Psychology Today, August 20, 2020. https://www.psychologytoday.com/us/blog/talking-about-trauma/202008/ayahuasca-and-its-potential-treat-ptsd
Palhano-Fontes, F., et al (2018). Rapid antidepressant effects of the psychedelic ayahuasca in treatment-resistant depression: A randomized placebo-controlled trial. Psychological Medicine, 49(4), 655-663. https://doi.org/10.1017/S0033291718001356
WHO. 2018b. Global health estimates: disease burden by cause, age, sex, by country and by region, 2000–2016 [online]. Geneva, Switzerland. www.who.int/healthinfo/global_burden_disease/estimates/en/index1.html
Tyrväinen, L., Ojala, A., Korpela, K., Tsunetsugu, Y., Kawaga, T. & Lanki, T. 2014. The influence of urban green environments on stress relief measures: a field experiment. Journal of Environmental Psychology, 38: 1–9.
Martens, D. & Bauer, N. 2013. Natural environments – a resource for public health and wellbeing. A literature review. In E. Noethammer, ed. Psychology of wellbeing: theory, perspectives and practice, pp. 173–217. New York, USA, Novas Science.
Li, Q. (2010). Effect of forest bathing trips on human immune function. Environmental Health and Preventive Medicine, 15(1), 9-17. https://doi.org/10.1007/s12199-008-0068-3
Li, Q., et al (2009). Effect of phytoncide from trees on human natural killer cell function. International Journal of Immunopathology and Pharmacology, 22(4), 951-959
Kobayashi, H., Song, C., Ikei, H., Park, B.-J., Kagawa, T., & Miyazaki, Y. (2019). Combined effect of walking and forest environment on salivary cortisol concentration. Frontiers in Public Health, 7, Article 376. https://doi.org/10.3389/fpubh.2019.00376
Ulrich RS. View through a window may influence recovery from surgery. Science. 1984 Apr 27;224(4647):420-1. doi: 10.1126/science.6143402. PMID: 6143402.