Bites from mosquitoes, ticks, flies, fleas, and lice are responsible for one of every six communicable diseases. Residential encroachment, inter-regional travel, and most notably, global warming increase vector-human encounters, putting 80% of the world’s population at risk. According to the World Health Organization (WHO), parasites, bacteria, and viruses transmitted by insect and arthropod vectors kill more than 700,000 people each year.
Chronic socio-economic, geographic, and environmental concerns and the repercussions of a flatter, expanding, and more connected society have allowed vector-borne diseases (VBDs) to continue to plague much of the world:
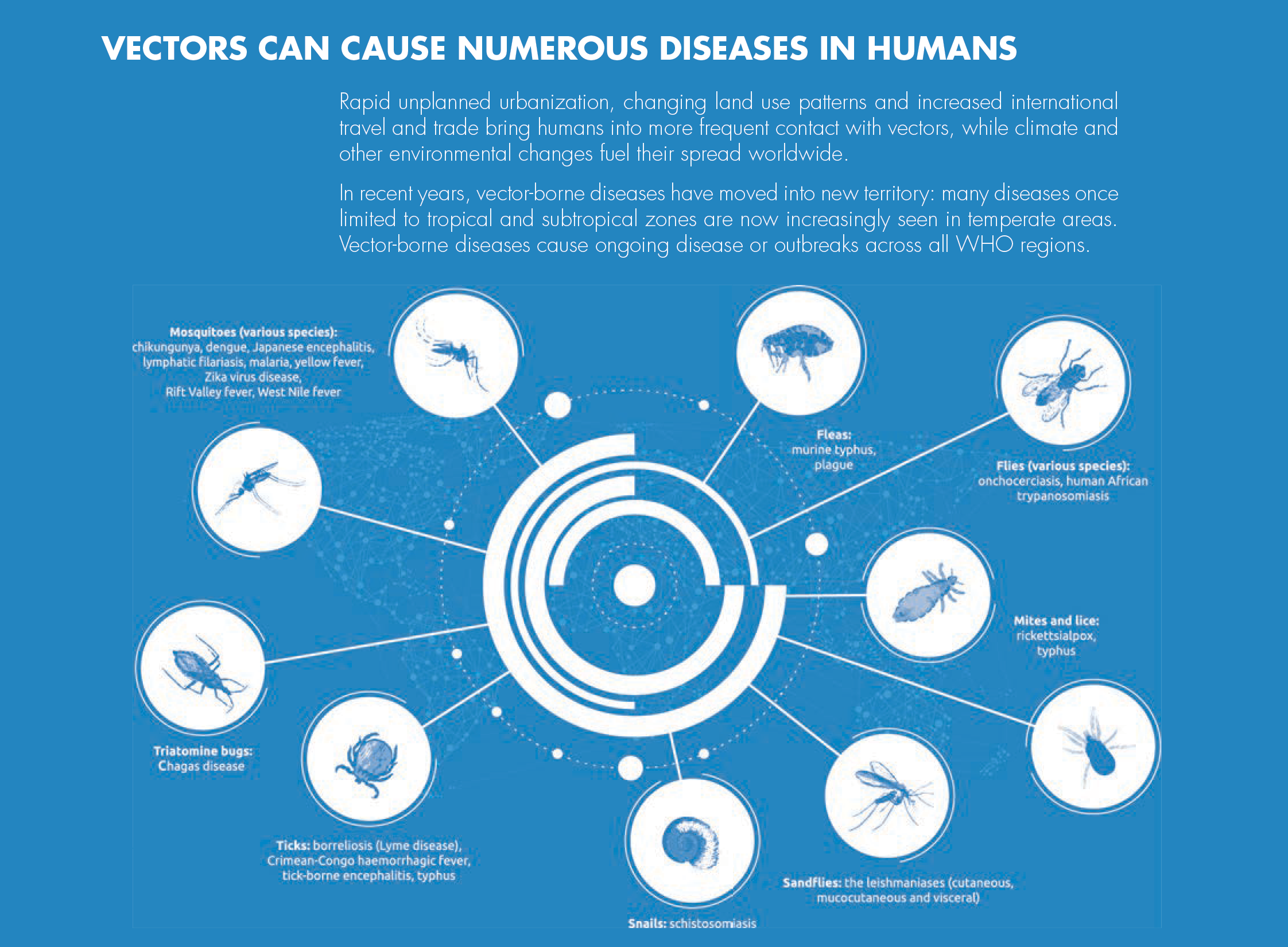
- Climate Change: Global warming makes more of the world habitable for vectors and extends their life cycles and breeding seasons.
- Urbanization: Denser populations foster disease spread and offer safe harbor and breeding grounds. Developments near woodlands and prairies bring people into greater contact with ticks, mosquitoes, and other vectors.
- Mobilization: Easy global travel and population migration allows VBDs to spread across regions and even continent to continent.
- Resource Inequality: Underdeveloped countries lack the resources to monitor outbreaks, control vectors, and treat patients.
Coupled with unreliable drinking water and poor excreta management in large swaths across the Global South, these factors have generated uncontained pandemics such as the 2016 Zika outbreak in Central and South America and the 2015 yellow fever resurgence in Angola the Democratic Republic of Congo.
These diseases, along with malaria, dengue, and others prevail in underdeveloped regions where poor nutrition exacerbates their effects and poverty hamstrings governments’ ability to respond.
Vector Control is Critical, but Challenging
The quest for relief from disease-carrying bugs goes back at least as far as the 5th century BC., when Herodotus recorded ancient Egyptians’ use of castor oil to repel mosquitoes. Romans, Greeks, and Native Americans also burned plant materials and rubbed oils on themselves to discourage vectors. These preventative measures led to other “vector-based interventions such as habitat management, insecticides, larvicides, bednets, and biological control [that] have successfully reduced the impact of most mosquito-borne diseases,” according to a report in Trends in Parasitology. “In many underdeveloped countries, traditional control methods like indoor residual spraying with chemical insecticides such as DDT and the use of pyrethroid-treated nets have been relied upon for vector control.”
A WHO report concludes that “vector control interventions have one of the highest returns on investment in public health,” While a 2020 study in PLOS Neglected Tropical Diseases notes that current-generation vector control “to a greater extent than drugs or vaccines, has been responsible for shrinking the map of many VBDs.”
Employed effectively, vector control not only reduces the infection rate but also contributes to non-health-related social and economic benefits such as greater productivity, gender equity, and poverty relief.
Unfortunately, large geographies remain troubled by VBDs due to limited funding that results in insufficient and poorly designed and executed intervention programs.
“There are numerous examples of where upsurges have resulted from weakening of control programs, particularly for malaria and dengue,” WHO laments. “The lack of sustainable and targeted financing has been underpinned by many factors, such as poor coordination within and between sectors, weak or non-existent monitoring and evaluation systems, and limited sustainable and proven interventions for certain vectors and situations….The result is that the full impact of vector control has not yet been achieved, even though this is often the best-proven or the only available preventive measure against most vector-borne diseases.”
The Need for Drone-based Control Methods
Achieving global vector control’s potential requires “realigning programs to optimize the delivery of interventions that are tailored to the local context [and]…strengthened monitoring systems and novel interventions with proven effectiveness.” This includes “integration of non-chemical and chemical vector control methods [and] evidence-based decision making guided by operational research and entomological and epidemiological surveillance and evaluation.”
Drones or “unmanned aerial vehicles” (UAVs) can save time and money compared to conventional ground-based surveys. Sophisticated models and monitoring equipment can be purchased for a few thousand dollars. They don’t require a pilot’s license, they are becoming easier to fly, and their paths can be fully automated through AI, machine learning, global positioning systems, and computer vision.
Drone technology continues to demonstrate an ability to address WHO’s call for integrated vector management that includes pesticide management, cost-effective measures, and environmental care that will “overcome challenges experienced with conventional single-intervention approaches to vector control; as well as recent opportunities for promoting multi-sectoral approaches to human health.”
Drones are uniquely positioned to maximize advances in biotechnology, remote sensing, artificial intelligence, global positioning, and other technologies that can be applied to vector control. Drones can be adapted to address all types of vector control methods, including chemical agents, biological intervention, and habitat modification at all stages of vector development – eggs, larvae, and adults.
Entomologists and epidemiologists exploit this versatility in several ways:
Surveillance, Tracking, and Monitoring
WHO has prescribed “the continuous and systematic collection, analysis and interpretation of disease-specific data and the use of those data in the planning, implementation and evaluation of public health practice [as] a core intervention” practice in in all malaria-endemic and malaria-susceptible countries. This mandate has found a powerful ally in drones, which are revolutionizing how the world monitors and responds to disease vectors across diverse environments.
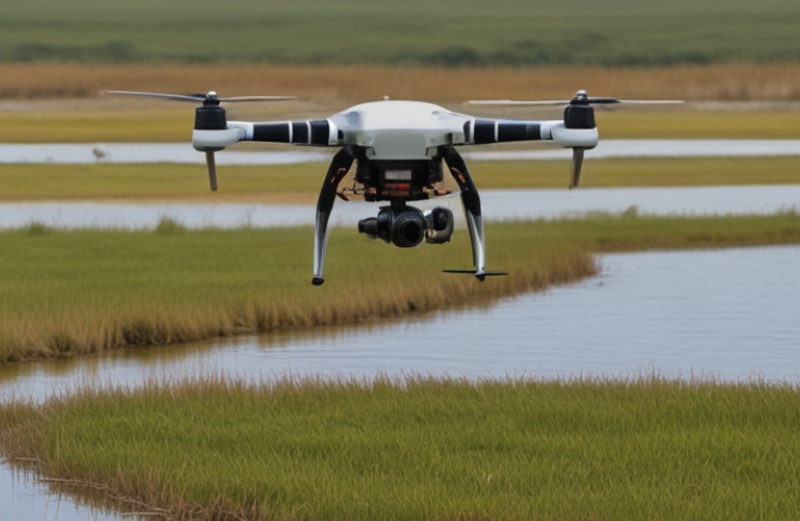
Nepad
Surveillance in the vector control context includes longitudinal studies of vector habitats to identify changes such as geologic shifts that create or destroy standing water sources and other breeding grounds; or an influx or outflux of humans or animals on whose blood vectors can feed. Inexpensive commercial-scale drones reduce the time and labor that must be invested to identify and sanitize the often-remote locations where vectors lay their eggs and where their larvae mature.
Unlike traditional ground units that may struggle to access remote or dangerous locations or disrupt the natural ecosystem, drones are highly maneuverable, so they easily navigate marshlands, dense forests, and flood-prone regions. Their mobility and overhead perspective enable operators to maneuver them around tree canopies, over bodies of water, through gulleys, and into other advantageous visual positions to capture comprehensive views of potential breeding sites.
They can easily transport deployable cargo and carry a diverse and impressive array of data-collecting equipment. Beyond standard visual imaging and video cameras, they can be equipped with multispectral sensors, temperature monitors, and even water salinity measurement capabilities. Sophisticated deployments can achieve spatial resolution of less than 10 centimeters per pixel, far surpassing the 20-meter standard from satellite imagery. While their coverage is limited to approximately one square kilometer per 30-minute flight, this exceptionally high resolution gives researchers and health policymakers the detail they need for precise habitat mapping and intervention planning. The images and other details inform the creation of up-to-date maps of vector infestations and breeding sites policy makers can use to determine which habitats to prioritize based on transmission risks, and which control methods will deliver the best results.
Drone surveillance has proven its mettle across diverse environments. In California’s tidal marshes, the Amazon basin, and Australia’s coastal saltmarshes, they have demonstrated greater than 80% accuracy in classifying potential vector breeding areas as shallow as 10 centimeters. These capabilities extend beyond simple visual identification – modern drone systems collect comprehensive environmental data, including water temperature variations, salinity levels, soil moisture content, and vegetation density.
Radar, LiDAR, and photogrammetry techniques that create three-dimensional terrain models promise to expand the role of drones in vector tracking and habitat monitoring that predict water flow, analyze vegetation growth and canopy coverage, and highlight undergrowth and human construction that may contain standing water where vectors can lay their eggs. The ability to detect changes in landscape features over time provides crucial information about emerging vector habitats.
Earth observation (EO) data from drones brings the potential to not only confirm putative breeding sites but also to categorize their size, depth, and composition. This could prove instrumental in selecting the most effective control methods.
According to one study, “the specific classes of interest are highly dependent on the local vector ecology. For example, An. gambiae breed in small or transient, often man-made, water bodies across a wide range of habitat types, including puddles on roads, agricultural irrigation…, sunlit rivers and streams, and quarries or construction sites. As these breeding sites are often temporary and may be difficult to directly observe…, identifying important land types where breeding sites occur can be an important proxy to target vector control measures.”
Policymakers increasingly rely on drone-collected data invaluable to formulate evidence-based decisions. Vector infestation and breeding site maps foster estimates of transmission risks so they can prioritize interventions. This use case emphasizes the criticality of consistent data collection practices and accurate interpretation. Cross-regional compatibility standards, regular equipment calibration procedures, and comprehensive operator training will ensure that data collected in different areas can be meaningfully compared and mobilized.
Automated image analysis and machine learning algorithms that identify breeding sites and recognize patterns that might escape human observation support real-time monitoring. With these capabilities, live data transmission to control centers and immediate alerts about new breeding sites become more feasible, enabling rapid response coordination for control measures.
Applications
Many of the same advantages drones possess in monitoring activities also apply to delivery of pesticides targeting adult mosquitoes and – more prevalent to date – larvicides introduced into standing water.
Drone developers have placed greater emphasis on agricultural uses for drone application of pesticides and herbicides, leaving the vector control sector to modify available products for their specific missions. These adaptations have been easier for surveillance than for payload delivery, but as the demand for pesticide-spraying drones increases, manufacturers can be expected to develop models specially designed for the tasks. Customized drones will incorporate greater payloads, miniaturized monitoring equipment, and calibrated sprayers – supercharging their effectiveness, speed, and cost-efficiency. Manufacturers have already created non-toxic sprayable liquids and granular pellets that can be dropped from drones.
Drone-based insecticide delivery also eliminates the environmental detriments caused by trucks and track-driven traffic in forests and manual spraying by people walking through wetlands. They present other benefits, as well:
- Precision: Unlike fixed-wing aircraft and helicopters, drones can be equipped with precision spraying and dispersal systems that can drop payloads in minutely targeted areas. This ensures minimal exposure to surrounding ecosystems and human settlements. Operators can use GPS mapping to map out predetermined routes and program drones to spray specific areas where disease vectors lurk.
- Economy: Flying at lower levels than is possible for crewed aircraft, drones’ payloads are less susceptible to being blown off course or deflected by trees, cellular towers, or utility lines. They need fewer chemicals because less is wasted, and more is concentrated where it will be most effective.
- Speed: Drones cover large areas more quickly and completely than human-operated spraying methods. They fly at consistent speeds and optimal altitudes, leading to faster eradication of vector populations and reduced labor costs. Operators on foot must cope with uneven terrain and inaccessible areas where they may not be able to get the spray coverage to be effective.
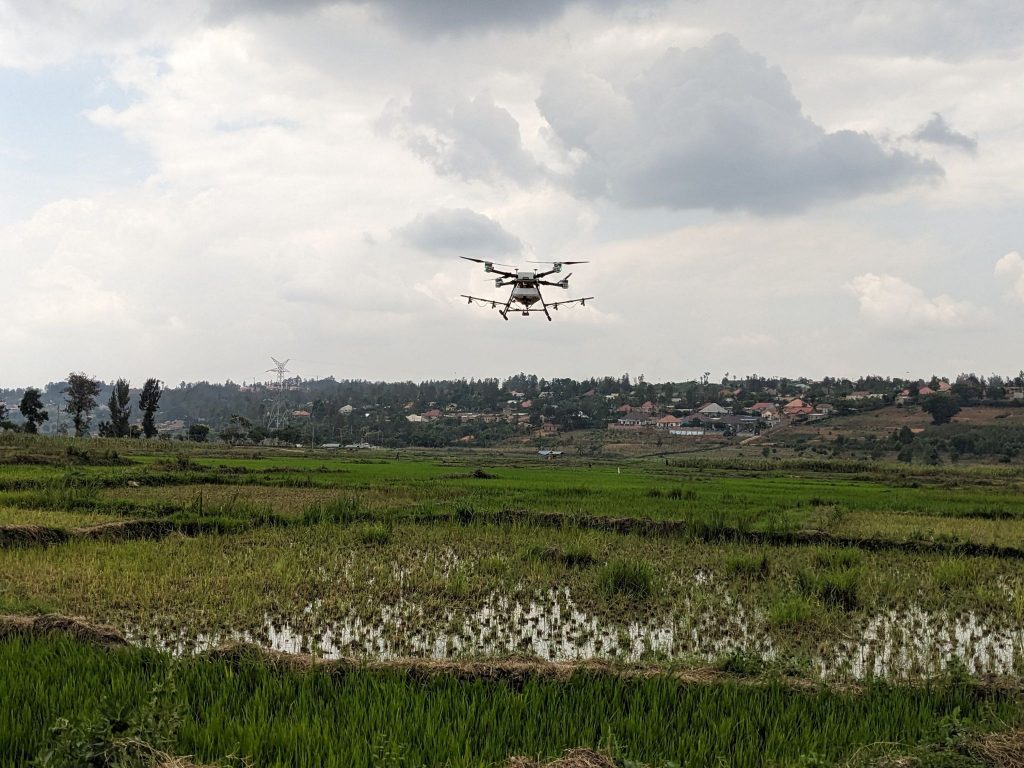
CHARIS UAS
Drones’ extensive use in locating and identifying vector breeding areas has led to their nascent use in deploying larvicides. Major successes, especially in Africa at the culmination of the Global Malaria Eradication Program, demonstrate that treating water bodies that are potential larval habitats remains a viable strategy. Recent experiments (See Case Study) provide evidence that larval source management (LSM) could be employed more extensively than current WHO recommendations which states that insecticides be placed in water sources only “in some specific locations, where vectors tend to breed in permanent or semi-permanent water bodies that can be readily identified.” The theory is that larvicide should merely supplement the use of indoor residual spraying (IRS) and long-lasting insecticidal nets (LLINs).
Advocates contend that more widespread LSM projects will help overcome the effects of outdoor vector biting behavior and insecticide resistance – two key contagion spreaders that LLIN and IRS cannot control.
Drones for Larviciding
The use of drones to apply larvicidal Bacillus thuringiensis var. israelensis (Bti) bacteria, a target specific option for controlling mosquitoes, is one of the most promising vector control options. A recent program in suburban Kigali, Rwanda used drones to both map perennial water bodies, temporary stagnant ponds, and human-flooded rice fields and to spray Bti in and among them.
According to the study, after 20 applications, mosquito larvae, pupae, and adult populations and the reported number of malaria cases all fell significantly.
“At the start of the larviciding intervention in July 2020, 72 cases of malaria were reported from the 12 contingent villages to intervention site with an incidence rate of 2.6 cases per 1000 inhabitants for a population of 27,254 people. In April 2021, at the end of the intervention, malaria cases dropped to 35 cases with 1.31 cases for 1000 inhabitants.”
“The scaling up of larviciding using drone technologies for prior mapping of potential mosquito breeding sites and then spraying of larvicide proved its effectiveness not only in targeted sites as recommended by the WHO but also in complex Anopheles breeding habitats,” the study authors reported. “These vector control technologies have to be integrated by malaria programs to enhance the gains in malaria control and elimination efforts.”
Case Study – Larvicide Surveillance for Malaria Control in Zanzibar, Tanzania
Researchers discovered that drones equipped with advanced sensors and software to collect high-resolution spatial data delivered significantly more accurate maps of potential mosquito breeding sites. While traditional ground-based mapping using people on foot and in vehicles found 48% of existing water bodies and 52% of those actually harboring mosquito larvae, drones employing a spatial intelligence system identified 69% of water bodies and 86% of those containing larvae.
Drones were especially good at mapping large water bodies like rice paddies (97% accuracy) and swamps (100% accuracy) and surveying flooded areas and dense vegetation that ground teams could not access easily. However, drones were not perfect; they found only 25% of small puddles and casual water accumulations (less than 2 meters wide) and 60% of sites hidden under tree canopies, particularly near villages, pointing to the need for multimodal surveillance and intervention.
Integrating drone mapping with smartphone technology allowed field workers to navigate to drone-identified sites, finding an additional 22% of water bodies during their treks.
While the drone operation project required a heavy initial investment to build trust through community engagement, it proved economical in the long run. Drone surveillance cut manual mapping labor by about one-third, from 1,603 hours 1,102 hours versus 1,603 hours for traditional mapping over six treatment rounds. Total cost averaged about $2 per person protected.
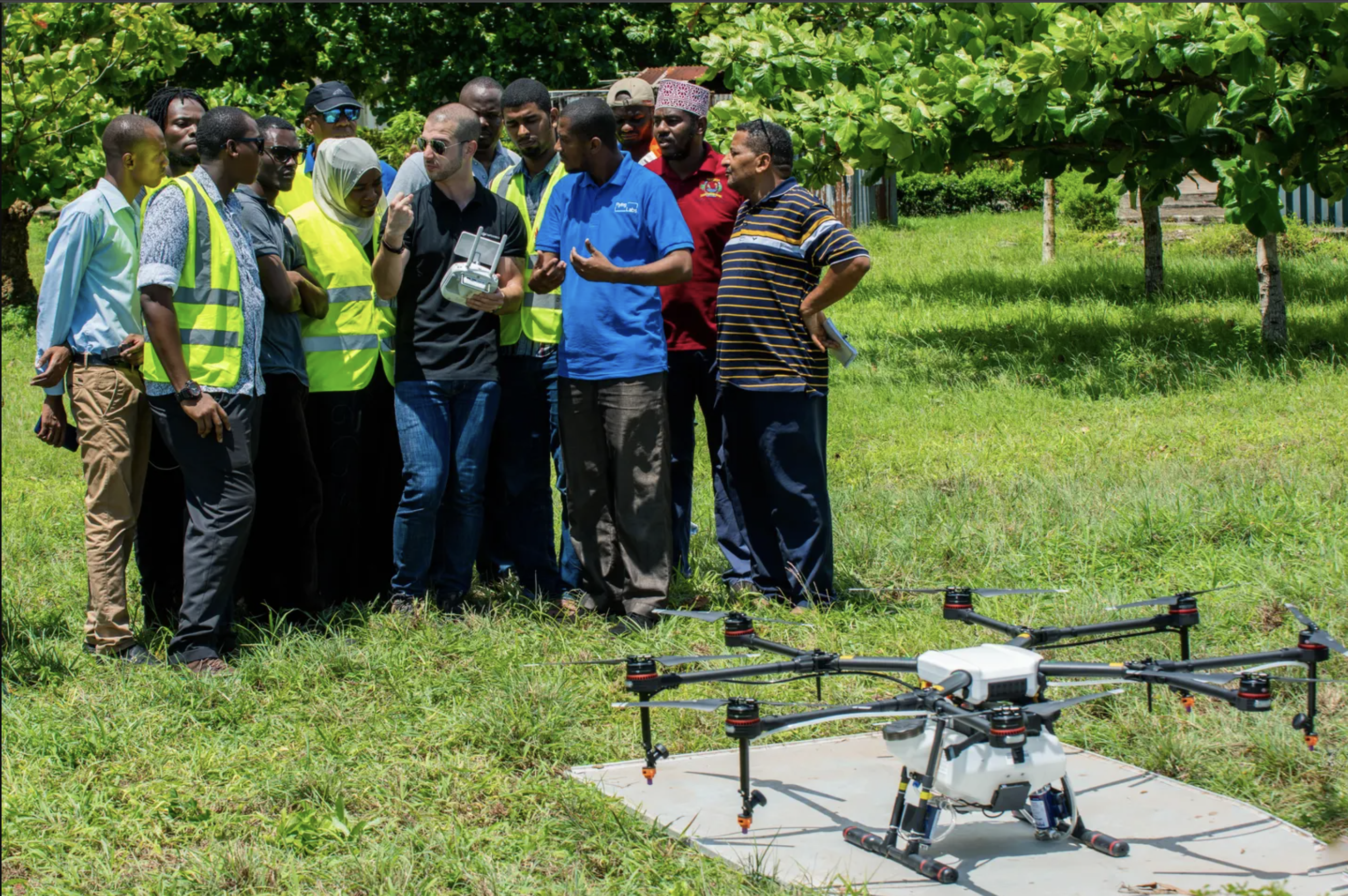
Tanzania Flying Labs
Drones for Adulticiding
Insecticide space spraying, the dispersal of a liquid ‘mist’ to kill adult insects is a well-established technique for vector control and in response to disease outbreaks. Where space spraying is utilized as a vector control mechanism, adulticide has been released via handheld, truck-mounted, or crewed aircraft equipment.
Drone-delivery of adulticides is just beginning to be explored, and few experiments have measured its effectiveness. One study saw a drone-delivered adulticide reduce the adult population of the dengue-carrying Aedes albopictus mosquito by 90%. Biting behavior also fell by 70% after 24 hours but returned to just a 9% reduction after 48 hours. These results must be repeatable and extendable over wide areas if they are to be useful as part of a comprehensive vector control program.
The modest adulticide spray rates needed to provide comprehensive coverage can be accomplished by drones; however, challenges, such as flight time/battery life and payload capacity, linger. Still, it is possible to adjust the larvicidal sprayers in use on drones to accommodate adulticide mists targeting tree canopies, refugee tents, and building surfaces where adult vectors rest. But to be truly effective the fog must comprise droplets small enough to remain airborne for several minutes, giving them time to contact mosquitoes while they are in flight.
Drones for Sterile Insect Technique (SIT)
Drones offer a scalable solution for dispersal of sterilized insects and insects modified through genetic techniques across vast and often inaccessible areas.
For instance, the World Mosquito Program (WMP) introduces the Wolbachia bacteria into native Aedes aegypti populations by releasing infected male and female mosquitoes into their midst. When uninfected females mate with infected males, their eggs will not hatch. WMP reported that “the use of aerial releases demonstrated comparable outcomes to ground-based releases [in terms of coverage area and disease spread] without the required labor or risk” associated with ground-release programs. Tests in Fiji used a cannister filled with 160,000 infected mosquitoes. The drone flew to more than 1,000 points, releasing about 150 individuals at each stop.
Conclusion
Drones clearly deserve a role in the global struggle against insect and arthropod disease vectors. While high winds, heavy rains, dense tree canopies, and other weather, geographic, and environmental factors limit universal applications, drones can operate below cloud cover that obstructs satellite surveillance and be launched in small clearings that crewed aircraft cannot negotiate, filling gaps in surveillance and ground-based chemical and biological control distribution.
Advances in monitoring technology, battery life, and payload capacity point to expanded reliance on UAVs, as manufacturers continue to explore use cases for monitoring and distributing biological solutions to control vectors. Drones’ precision, maneuverability, and low operating costs will prove particularly beneficial in eradicating and replacing vector populations in concentrated transmission environments and across difficult terrain.
As the technology gains wider applications and acceptance among at-risk populations, environmental stewards, and airspace regulators, the international community must come together to develop and enforce best practices regarding privacy and safety. Additional studies should be performed to quantify UAV’s advantages in vector control initiatives.
By Scott Smith